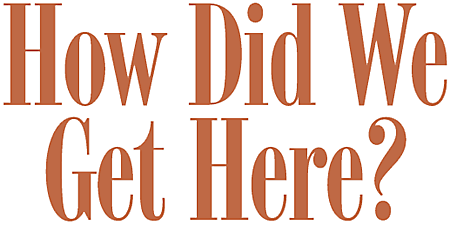
Astronomers cannot see all the way back in time to the origin of the universe, but by drawing on lots of clues and theory, they can imagine how everything began.
Their model starts with the entire universe as a very hot dot, much smaller than the diameter of an atom. The dot began to expand faster than the speed of light, an expansion called the Big Bang. Cosmologists are still arguing about the exact mechanism that may have set...